Fusion energy—the power of the sun bottled up on Earth. It’s hailed as clean, limitless, and potentially the solution to humanity’s energy and climate crises. But is it all too good to be true? Could this “holy grail” of energy science just be an expensive mirage, or worse, a massive money pit? Let’s unravel this.
The Great Promise of Fusion
For decades, scientists and engineers have chased the dream of controlled nuclear fusion. Imagine a world where energy is as abundant as sunlight, and power plants generate electricity without polluting the atmosphere or producing radioactive waste. Fusion energy promises a future free of fossil fuels, clean air, and practically endless energy.
The idea is straightforward: replicate the process that powers stars like our sun. In stars, hydrogen nuclei (protons) fuse together under immense pressure and temperature, releasing colossal amounts of energy. On Earth, this means creating a mini-sun in a controlled environment.
It sounds easy in theory. In practice? Not so much.
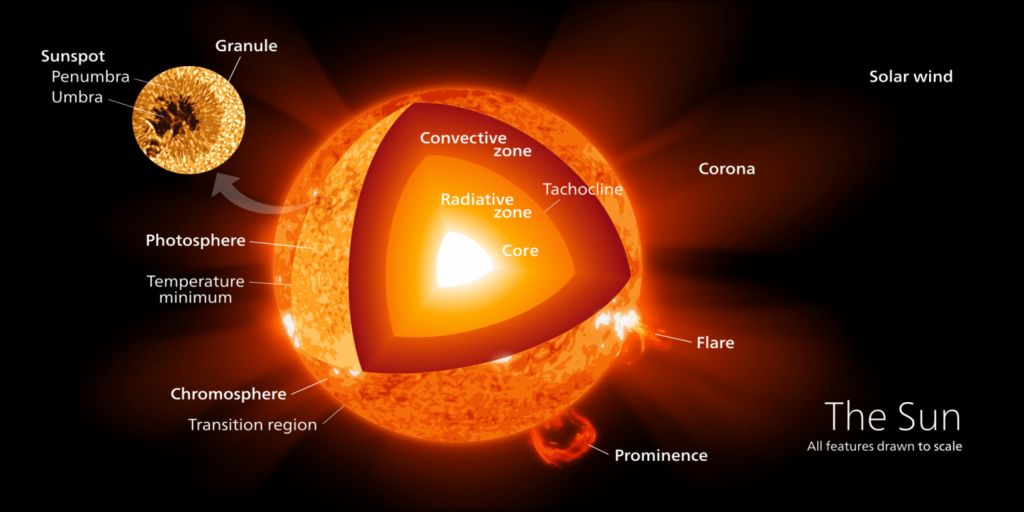
The Science of Fusion: Tiny Cores, Massive Energy
To appreciate fusion, we must first zoom into the atomic nucleus. Atoms consist of a nucleus (protons and neutrons) surrounded by a cloud of electrons. If an atom were the size of a sports stadium, the nucleus would be a tiny rice grain at the center. And yet, this minuscule core is packed with tremendous energy.
The force that holds atomic nuclei together—called the strong nuclear force—is one of the most powerful forces in the universe. But here’s the catch: to release that energy, you must either split large nuclei apart (fission, like in today’s nuclear power plants) or fuse smaller ones together (fusion). Both processes unlock the immense energy trapped within the nucleus.
Fusion holds a particular allure because it’s the most efficient way to harness nuclear energy. In fission, heavy elements like uranium are split, releasing energy. Fusion, on the other hand, involves combining light elements like hydrogen to form helium, releasing even more energy per reaction. It’s the ultimate cosmic recycling program.
The Fusion Goldmine: Hydrogen to Helium
Fusion energy draws its power from the simplest element in the universe: hydrogen. Hydrogen comes in a few flavors, the most important for fusion being deuterium (hydrogen with one neutron) and tritium (hydrogen with two neutrons). When deuterium and tritium nuclei collide and fuse, they produce helium and a tremendous amount of energy.
Sounds simple, right? But achieving fusion is a Herculean challenge. The sun accomplishes this feat with its massive gravity, squeezing hydrogen nuclei together at pressures and temperatures unimaginable on Earth—around 250 billion atmospheres and 15 million degrees Celsius.
On Earth, we lack the sun’s gravity. Instead, scientists must generate those conditions artificially, which means heating hydrogen gas into a plasma—a state where electrons and nuclei are no longer bound together—and confining it long enough for fusion to occur.
The Engineering Marvel of Fusion Reactors
Creating a controlled fusion reaction requires sophisticated technology. Scientists rely on magnetic fields to trap the plasma—a searing soup of charged particles. The most promising design for a fusion reactor is the tokamak, a doughnut-shaped device that uses magnetic fields to contain and compress the plasma.
Tokamaks are awe-inspiring but notoriously challenging. The plasma is unstable, constantly trying to escape confinement. And maintaining the necessary conditions for fusion—temperatures of over 100 million degrees Celsius—requires an extraordinary amount of energy and precision.
Europe’s flagship tokamak, the ITER project in France, is the largest and most ambitious fusion experiment to date. Planned in the 1980s, under construction since 2010, and scheduled for completion in the late 2030s, ITER aims to demonstrate that fusion can produce more energy than it consumes—a feat yet to be achieved.
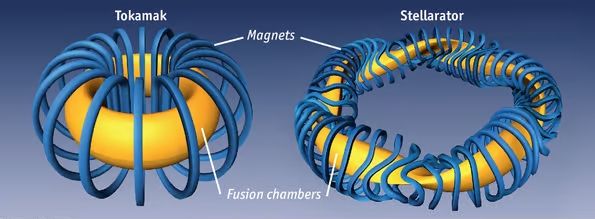
The Competition: Tokamaks versus Stellarators
While tokamaks dominate fusion research, another contender has emerged: the stellarator. Unlike tokamaks, which rely on internal plasma currents to sustain magnetic fields, stellarators use a complex network of external magnets. This design avoids the instability issues of tokamaks and could, in theory, sustain continuous plasma confinement.
Germany’s Wendelstein 7-X, a cutting-edge stellarator, is leading the charge in this area. With its intricate magnetic field design, it holds the promise of a steady-state fusion reactor—something tokamaks struggle to achieve.
Fusion’s Long Road: Is it Science or Fantasy?
Critics often point out the seemingly endless delays and ballooning budgets of fusion projects. “Fusion is always 30 years away,” they quip. And it’s true—fusion energy has been the great “almost” of energy science for decades.
But calling fusion a failure misses the point. It’s not that scientists don’t know how to achieve fusion. The problem is doing it efficiently, affordably, and reliably. Fusion is one of the most complex engineering challenges humanity has ever faced. It requires a level of precision and innovation that pushes the boundaries of what’s possible.
It’s worth remembering that today’s technologies—space travel, advanced computing, even the humble smartphone—once seemed like unattainable dreams. Fusion may take longer than we’d like, but the rewards are too great to ignore.
The Climate Crisis
Some argue that fusion won’t arrive in time to combat the climate crisis. They’re right—fusion is unlikely to replace fossil fuels in the next two decades. But that doesn’t diminish its importance.
Fusion is about the long game. It’s about building an energy system that can sustain humanity for millennia, not just decades. As the world shifts toward renewable energy sources like wind and solar, fusion could eventually provide the steady, baseload power needed to complement these intermittent sources.
In a world where energy demands continue to grow, fusion offers a path to near-limitless power without greenhouse gas emissions or radioactive waste.
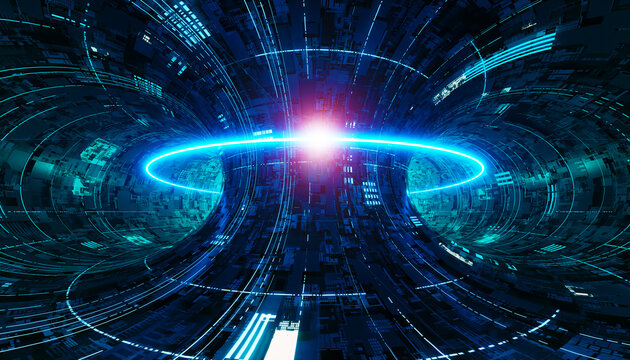
Is Fusion Worth the Cost?
Fusion research is expensive—ITER alone has a price tag of over $20 billion. But when you consider the potential payoff, the cost seems almost trivial. A single liter of seawater could provide enough deuterium to produce as much energy as burning a barrel of oil. With fusion, humanity could unlock an energy source that lasts billions of years.
Moreover, fusion research drives innovation in fields like materials science, plasma physics, and superconducting technology. Even if commercial fusion reactors are decades away, the knowledge gained is invaluable.
The Path Forward
Fusion may be the energy source of the future, but it’s a future that requires patience, investment, and global collaboration. ITER, Wendelstein 7-X, and other projects represent humanity’s determination to tackle one of the universe’s most profound challenges.
Fusion is not just about energy. It’s a symbol of what we can achieve when we dare to dream big and push the limits of science and engineering. It’s a reminder that progress often comes slowly, but the rewards are worth the wait.
So, is fusion the salvation we’ve been waiting for or an expensive illusion? The truth lies somewhere in between. What’s clear is that fusion represents a promise—a promise of a cleaner, brighter, and more sustainable future. And that’s a promise worth keeping.