It’s a strange time in the cosmos. On one hand, we have the Lambda-CDM model, our golden standard for understanding the universe, combining the cosmological constant (Lambda) with cold dark matter (CDM). It’s been our reliable guide for explaining everything from the expansion of the universe to the large-scale structure of galaxies. Yet, as much as we love this model, there’s a nagging problem: we still haven’t directly found the ingredients.
Dark energy? Still a mystery. Cold dark matter? Also missing in action. It’s like we’ve baked a cake, but no one has ever actually seen the flour. Sure, the cake tastes great, but something is starting to feel a little off. And now, as our cosmic cake continues to crumble, it seems the universe might be in crisis. Buckle up, we’re diving into the wild world of cosmology’s most baffling conundrums.
Once Upon a Time: Simpler Days in the Universe
There was a time when cosmology was more straightforward—or so it seemed. The universe was expanding, and everything was illuminated by stars and galaxies. Simpler times, right? But as we’ve dug deeper into the mysteries of the universe, we’ve realized things are far more complicated. We now know that dark energy is driving the accelerated expansion of the universe, and dark matter—or more specifically, cold dark matter—is holding galaxies together in ways that visible matter alone simply can’t explain.
The story of dark matter begins in 1935 when astronomer Fritz Zwicky noticed something strange about galaxy clusters. The galaxies were moving so fast that, by all rights, they should have flown apart. There wasn’t enough visible matter to keep them gravitationally bound. Zwicky proposed that there must be some invisible mass—something that wasn’t shining like stars but was still exerting gravitational force. This was the birth of the dark matter hypothesis.
The Cosmic Detective Work Begins
As the years passed, more evidence for dark matter piled up. Astronomers measured the rotation curves of spiral galaxies, expecting them to behave like the planets in our solar system: the farther from the center, the slower things should move. But that wasn’t the case. The stars at the outer edges of galaxies were moving just as fast as those near the center. This could only be explained by the presence of extra, invisible matter—five to six times more than the visible stuff.
Then came gravitational lensing. According to Einstein’s general relativity, massive objects bend spacetime, and this curvature can warp the paths of light traveling through space. If there were large amounts of dark matter, they should act like cosmic magnifying glasses, distorting the light from distant galaxies. And guess what? We see this exact phenomenon, confirming that something heavy and invisible is lurking out there, warping space like a giant, unseen hand.
The Rise of Cold Dark Matter
So, we know that dark matter exists, but what kind of matter is it? The leading theory has long been that it’s cold dark matter (CDM)—particles that are slow-moving and heavy enough to form the small gravitational wells that galaxies and clusters need to grow. Cold dark matter explains how galaxies like our Milky Way formed, and why structures in the universe look the way they do.
Without cold dark matter, we wouldn’t have the universe we see today. In fact, galaxies couldn’t even form! In the early universe, visible matter was so tightly coupled to radiation that it couldn’t clump together. But dark matter, being invisible to light, could quietly form the seeds of galaxies, allowing visible matter to eventually gather and create the structures we see.
But here’s the catch: we still haven’t found cold dark matter.
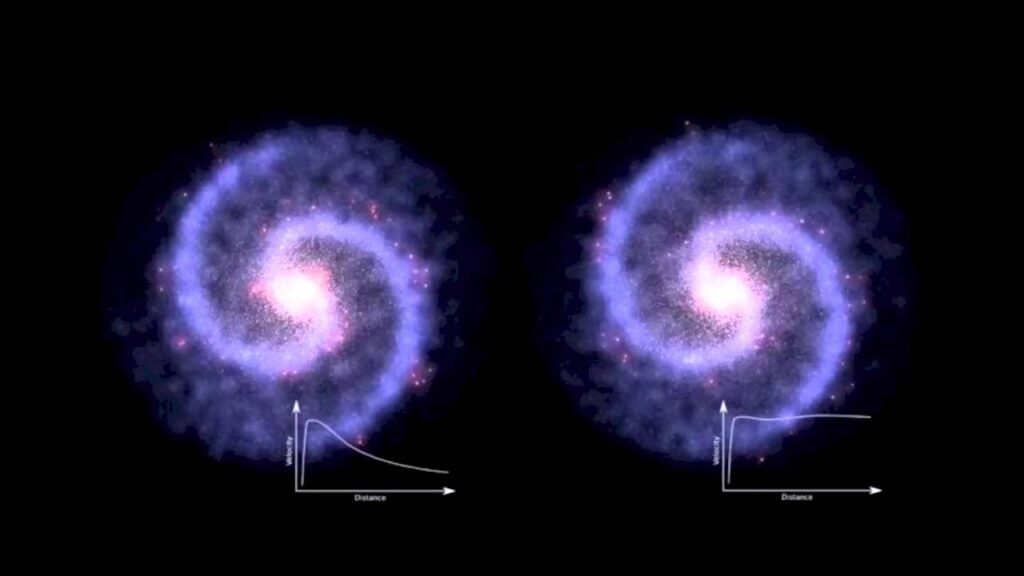
Searching for the Invisible: WIMPs and MACHOs
Physicists have spent decades trying to detect dark matter, focusing on particles called WIMPs (Weakly Interacting Massive Particles). These hypothetical particles should only interact with regular matter through gravity and the weak nuclear force, making them nearly impossible to detect—except through some clever tricks.
Experiments have been set up to look for WIMP collisions with atomic nuclei, which could produce detectable signals. The theory goes that when a WIMP bumps into a regular atom, it should cause a tiny burst of energy, like a cosmic tap on the shoulder. But so far, no dice—WIMPs have remained frustratingly elusive.
Then there were the MACHOs (Massive Compact Halo Objects)—dark objects like black holes, neutron stars, or cold, dim stars that could make up the missing mass. But MACHOs can’t explain the full picture either. There simply aren’t enough of them to account for the vast amounts of missing matter in the universe.
And now, physicists are throwing out even more creative ideas, like RAMBOs (Robust Associations of Massive Baryonic Objects), groups of dead stars or dim white dwarfs huddled together in dark corners of the universe. But despite the imaginative names, none of these theories have panned out.
The Gravitational Crisis
It’s not just dark matter that’s causing us headaches. Dark energy, the mysterious force driving the accelerated expansion of the universe, remains just as puzzling. Dark energy makes up about 70% of the universe, and yet we still don’t know what it is. Some think it could be related to Einstein’s cosmological constant, a fixed energy that pervades space, while others speculate it might involve exotic particles or fields we haven’t discovered yet.
There are also those who suggest that maybe our understanding of gravity itself is flawed. Could Einstein’s general relativity, the cornerstone of modern physics, be incomplete? Perhaps we need a modified theory of gravity to explain these cosmic anomalies.
But here’s the kicker: despite all the evidence supporting dark matter and dark energy, we’ve yet to pin down exactly what they are. It’s like playing a game of cosmic whack-a-mole, where every new observation raises more questions than answers.
Why Lambda-CDM Might Be in Trouble
So where does that leave our beloved Lambda-CDM model? Well, the model still explains a lot—it’s the best we’ve got for understanding the universe on large scales. But it’s starting to look a little shaky. The recent dark matter detection experiments haven’t delivered the results we hoped for. Projects like the LHAASO (Large High Altitude Air Shower Observatory) in China, which measures cosmic and gamma rays to try and detect dark matter, have found nothing—not even a whisper of those elusive WIMPs.
And it’s not just the detection experiments. The whole idea of cold dark matter is starting to feel wobbly. Some scientists are even suggesting we might be dealing with lukewarm dark matter—particles that aren’t quite as slow-moving as CDM but not fast enough to disrupt galaxy formation. It’s the cosmic equivalent of a lukewarm cup of coffee: not too hot, not too cold, and definitely not what we ordered.
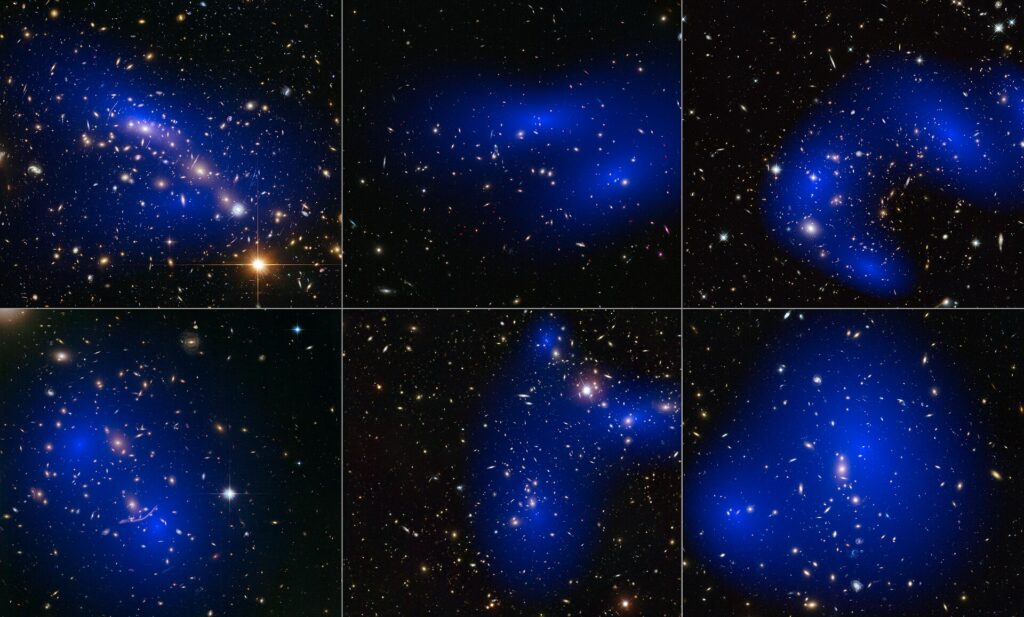
What If It’s All Wrong?
There’s even more desperation in the air when you hear some of the latest proposals. Some physicists are suggesting that primordial black holes from the early universe could account for dark matter. But how exactly do you create black holes in the first few seconds after the Big Bang? Your guess is as good as mine, but the idea is gaining some traction in the scientific community.
Others are re-examining modifications to Newtonian dynamics—essentially tweaking Newton’s laws to explain the strange behavior we’re seeing in galaxy rotation curves. But this would be a massive shift in our understanding of physics, and it doesn’t solve the problem of dark matter on larger cosmic scales.
Amidst all this uncertainty, there’s still some hope in the form of WIMPs and interacting massive particles. These particles, if found, could offer the breakthrough we need. WIMPs don’t interact with light, making them hard to detect, but if they occasionally bump into an atomic nucleus, we could catch them in the act. There’s also the possibility that dark matter particles could decay, releasing gamma rays that we might be able to detect.
Experiments like LHAASO and others are searching for these gamma rays, hoping to catch a glimpse of dark matter in action. So far, the results have been disappointing, but the search continues.
The Great Cosmic Mystery Continues
As it stands, the Lambda-CDM model remains our best description of the universe—but it’s on thin ice. We still don’t know what dark matter or dark energy actually are, and recent experiments haven’t brought us any closer to the answers.
It’s a bit like living in a detective novel where the suspects are all invisible, and the clues are scattered across billions of light-years. But that’s the beauty of science. Each new observation, whether it confirms our theories or shatters them, brings us one step closer to the truth.
So, while the cosmic crisis deepens and we continue to wrestle with the mysteries of the universe, one thing is certain: there’s a lot more to discover, and the adventure is far from over. Keep your telescopes ready, because the universe always has a few more surprises in store.